Longevity's Unsung Heroes: The Vital Role of Model Organisms in Aging Research
- Hussein Elwan
- Nov 27, 2023
- 8 min read
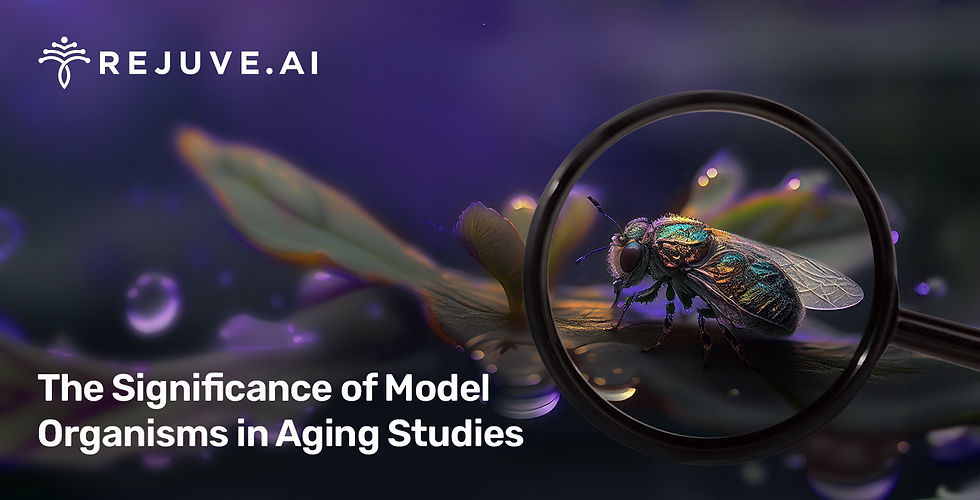
Behind the scenes of ethical debates and raised societal eyebrows, scientists certainly find no pleasure in conducting studies on non-consenting creatures.
Yet, from this controversy emerges a glaring truth: plenty of these studies have led to pivotal biomedical discoveries. From 2000 to 2017, a staggering 78% of Nobel Prizes in Physiology or Medicine traced their roots back to studies involving these unsung organisms [1].
When it comes to longevity science, they play an instrumental role as well, revealing numerous clues into why we age, how we do so, and what we can do about it.
So gear up, for today we’re taking a journey through the complex workings of model organisms in aging research.
Model Organisms: A Journey Through Aging Research History
By definition, model organisms are any non-human species that scientists study to understand specific biological phenomena and processes. The idea behind them is that researching these organisms generates knowledge that could then be projected into new domains [2].
It could be argued that the first use of model organisms dates back to ancient Greek civilizations, before the concept as we know it today was developed, when polymaths such as Aristotle experimented on animals [3]. However, it was thousands of years later in 1935 that model organisms made their way to aging research when researchers studied the effects of caloric restriction on life expectancy in rodents [4].
Since then, scientists have been performing similar experiments to study aging in a plethora of other species. Understandably, these studies are more feasible with model organisms than humans, given the ethical considerations, practical constraints, and extended lifespan, which pose challenges for conducting analogous experiments in our species [2].
Which Model Organisms Are Used to Study Aging?
AnAge, the Animal Ageing and Longevity Database, meticulously catalogs studies on aging in a remarkable array of 4,645 species, spanning mammals of all sizes, birds, fish, invertebrates, plants, and even bacteria [5].
The most commonly used organisms among these are yeast, a species of roundworms called C. elegans, fruit flies, and lab mice [6]. Typically, what scientists look for in an ideal aging model are a short lifespan (though some research ventures are more focused on longer-lived species), a well-characterized genome similar to humans, and practical ease in culturing and maintenance [7]. These criteria ensure efficiency in studying aging and extracting valuable insights for longevity science.
The Different Ways Model Organisms Help Us Understand Aging
Within this highly prolific realm of aging research, scientists have enlisted the help of model organisms in studies of various designs and objectives to comprehensively explore the intricacies of aging and longevity. The discoveries from many of these studies have been nothing short of transformational. So let’s delve into the distinct ways in which model organisms have been crucial in advancing our knowledge of aging.
Comparative Aging Studies
Comparative aging is a research approach that seeks to understand the mechanisms of aging by comparing the lifespans of different species [8]. For example, in mammals, the variation is striking, from the bowhead whale's remarkable 211 years to the brief six months to one-year lifespan of most mice species [9].
You can already guess the major factor behind this discrepancy. Yes, that would be size. Size unsurprisingly has major say over life expectancy, as it affects cell number, heart rate, metabolic rate, reproductive rate, and other critical parameters linked to survival [10].
However, interestingly enough, size doesn’t tell the full story. The Brandt bat, despite being a quarter the size of a mouse, boasts a 40-year lifespan [9], hinting at the role of factors like flight in evading threats.
Delving into all these factors and how they affect lifespan across different species not only generates fascinating insights about the interplay between evolution and biological aging, but also unlocks new avenues of research into human longevity.
Identification of Longevity Pathways
Various longevity pathways have been identified and for that, we have to thank our model organisms. The first of these pathways was discovered over two decades ago in C. elegans.
Researchers found that mutations in specific C. elegans genes doubled the lifespan of these worms, leading to the discovery of the insulin/insulin-like growth factor-1 (IGF-1) signaling pathway in aging regulation. Reduced insulin signaling in this pathway has shown promise in reducing the production of reactive oxygen species (ROS), which cause age-related damage in nucleic acids, proteins, and lipids [11].
These findings transcended to humans, highlighting the evolution of this pathway from a single receptor in invertebrates to more intricate networks in mammals. Notably, it was found that this pathway influences peripheral glucose metabolism and body fat distribution [12].
Studying Age-Related Damage Resistance Mechanisms
Most researchers agree that aging is caused by the accumulation of damage over an individual’s lifespan. Extraordinarily, one organism shows exceptional resistance to such age-related damage: the naked mole rat.
Hailing from East Africa, these rats live up to 30 years, a lifespan far exceeding that of other rodents, and maintain robust health throughout their lives. One of their strategies to achieve that is how they respond to senescence. Senescence refers to a state where cells lose their ability to divide and function effectively, which is a hallmark of aging. Naked mole rats have a unique natural ability to clear out these senescent cells. In other words, their bodies boast intrinsic senolytic mechanisms, which contribute to their longevity and healthspan [13].
Moreover, these creatures have an almost complete resistance to cancer. In a recent breakthrough, a gene associated with the naked mole rat’s cancer resistance was introduced into mice. This intervention resulted in improved health and extended lifespan in the mice—a groundbreaking leap in our understanding of aging and resilience [14].
Studying Age-Related Diseases
Model organisms serve as powerful tools in the study of age-related diseases. In Alzheimer’s disease research, for example, these organisms, which do not naturally develop Alzheimer’s, are genetically engineered to carry human genes associated with the disease. This allows scientists to observe the progression of Alzheimer’s in a controlled setting.
One of the key insights gleaned from these studies is the role of beta-amyloid plaques. These are clusters of protein fragments that build up in the brains of Alzheimer’s patients. Genetically modified mice have been instrumental in studying these plaques, as they accumulate in the brains of these mice in a manner similar to human patients [15].
Another significant discovery came from studying fruit flies. These tiny organisms have helped scientists identify several genes that influence the toxicity of Tau, a protein that forms tangles in the brains of Alzheimer’s patients. These tangles make another hallmark of Alzheimer’s disease [16].
These discoveries illuminate the critical contribution of model organisms in unraveling the mysteries of Alzheimer’s disease and other age-related conditions.
Testing New Longevity Interventions
This use case of model organisms is probably the one the general public is most familiar with. Model organisms are essential in pre-clinical trials of longevity interventions just like they are for any other type of intervention.
So to add some excitement, let's spotlight a recently launched and truly unique example of such studies: The Test of Rapamycin In Aging Dogs clinical trial. [17].
Dogs, beloved companions to many, aren't your typical model organisms. Additionally, the study's uniqueness extends to its dual purpose – not only developing interventions for humans but also for dogs, who get to call dibs on this treatment regimen if the trial succeeds before human trials are initiated.
The clinical trial involves a one-year regimen of weekly low-dose rapamycin, a compound previously demonstrated to extend lifespan and enhance health in mice. This trial seeks to assess whether rapamycin can extend lifespan, enhance heart and cognitive function, and decrease age-related diseases in middle-aged, large-breed dogs. Notably, it represents the first clinical trial outside a laboratory setting utilizing lifespan and healthspan metrics as endpoints, ushering in a new era in longevity research.
The New Era of Model Organisms in Aging, Powered by AI!
Unfortunately, in the admirable pursuit of breakthroughs in aging research, scientists face discouraging translational research outcomes, with frustrating failure rates of 90% for cancer [18], 99% for Alzheimer's [19], and 99% for stroke [20]. A resounding shift is imperative to build that bridge from laboratory discoveries to human advancements.
And this is exactly where visionary ventures like Rejuve Network’s Methuselah Flies Project shine!
Operating through two arms, Rejuve.Bio and Rejuve.AI, the former focuses on Methuselah Flies, selectively bred for longevity. Living 4.5 times longer than their peers, these flies reveal secrets crucial to aging [21]. Rejuve.AI steps in, leveraging one-of-a-kind AI models to analyze Methuselah Fly data, integrating it with human health and omic data. This transformative approach significantly accelerates drug discovery, propelling the translation of findings from flies to potential human applications.
The Methuselah Flies Project, with its built-in translational research mode, embodies a pioneering stride into a new era of aging research, powered by the fusion of biology and cutting-edge AI!
References:
[1] Galas, S. (2021). Animal Models of Aging. Springer EBooks, 448–453. https://doi.org/10.1007/978-3-030-22009-9_34
[2] Ankeny, R. A., & Leonelli, S. (2020, November 1). Model Organisms. Elements in the Philosophy of Biology. https://www.cambridge.org/core/elements/model-organisms/F895B26EAC0373BCA5A138835AC73AEA
[3] Hajar, R. (2011). Animal testing and medicine. Heart Views, 12(1), 42. https://doi.org/10.4103/1995-705x.81548
[4] Zainabadi, K. (2018). A brief history of modern aging research. Experimental Gerontology, 104, 35–42. https://doi.org/10.1016/j.exger.2018.01.018
[5] AnAge Database Statistics. (n.d.). Genomics.senescence.info. Retrieved November 21, 2023, from https://genomics.senescence.info/species/stats.php
[6] Valenzano, D. R., Aboobaker, A., Seluanov, A., & Gorbunova, V. (2017). Non‐canonical aging model systems and why we need them. The EMBO Journal, 36(8), 959–963. https://doi.org/10.15252/embj.201796837
[7] Holtze, S., Gorshkova, E., Braude, S., Cellerino, A., Dammann, P., Hildebrandt, T. B., Hoeflich, A., Hoffmann, S., Koch, P., Terzibasi Tozzini, E., Skulachev, M., Skulachev, V. P., & Sahm, A. (2021). Alternative Animal Models of Aging Research. Frontiers in Molecular Biosciences, 8, 660959. https://doi.org/10.3389/fmolb.2021.660959
[8] Austad, S. N. (2009). Comparative Biology of Aging. The Journals of Gerontology Series A: Biological Sciences and Medical Sciences, 64A(2), 199–201. https://doi.org/10.1093/gerona/gln060
[9] Longevity Records: Life Spans of Mammals, Birds, Amphibians, Reptiles, and Fish. (2022). Demogr.mpg.de. https://www.demogr.mpg.de/longevityrecords/0203.htm
[10] Speakman, J. R. (2005). Body size, energy metabolism and lifespan. The Journal of Experimental Biology, 208(Pt 9), 1717–1730. https://doi.org/10.1242/jeb.01556
[11] Kaletsky, R., & Murphy, C. T. (2010). The role of insulin/IGF-like signaling in C. elegans longevity and aging. Disease Models & Mechanisms, 3(7-8), 415–419. https://doi.org/10.1242/dmm.001040
[12] Barzilai, N., Etgen, A., Muzumdar, R., Scott Neal-Perry, G., Pessin, J., & Suh, Y. (n.d.). IGF-1 and Insulin Signaling Pathways in Aging | Institute for Aging Research | Albert Einstein College of Medicine. Www.einsteinmed.edu. Retrieved November 21, 2023, from https://www.einsteinmed.edu/centers/aging/research/igf-1-insulin-signaling-pathways.html
[13] Kawamura, Y., Oka, K., Takashi Semba, Takamori, M., Sugiura, Y., Yamasaki, R., Suzuki, Y., Takeshi Chujo, Nagase, M., Oiwa, Y., Fujioka, S., Homma, S., Yamamura, Y., Shingo Miyawaki, Narita, M., Fukuda, T., Sakai, Y., Ishimoto, T., Kazuhito Tomizawa, & Makoto Suematsu. (2023). Cellular senescence induction leads to progressive cell death via the INK4a‐RB pathway in naked mole‐rats. The EMBO Journal. https://doi.org/10.15252/embj.2022111133
[14] Zhang, Z., Tian, X., Lu, J. Y., Boit, K., Ablaeva, J., Zakusilo, F. T., Emmrich, S., Firsanov, D., Rydkina, E., Biashad, S. A., Lu, Q., Tyshkovskiy, A., Gladyshev, V. N., Horvath, S., Seluanov, A., & Gorbunova, V. (2023). Increased hyaluronan by naked mole-rat Has2 improves healthspan in mice. Nature, 1–10. https://doi.org/10.1038/s41586-023-06463-0
[15] For Alzheimer’s studies, animal model selection is everything. (n.d.). Www.nature.com. Retrieved November 20, 2023, from https://www.nature.com/articles/d42473-022-00118-w
[16] Prüßing, K., Voigt, A., & Schulz, J. B. (2013). Drosophila melanogaster as a model organism for Alzheimer’s disease. Molecular Neurodegeneration, 8(1), 35. https://doi.org/10.1186/1750-1326-8-35
[17] Dog Aging Project goal is to help both dogs and humans live longer, healthier lives. (2022, February 24). National Institute on Aging. https://www.nia.nih.gov/news/dog-aging-project-goal-help-both-dogs-and-humans-live-longer-healthier-lives
[18] Mak, I. W., Evaniew, N., & Ghert, M. (2014). Lost in translation: animal models and clinical trials in cancer treatment. American Journal of Translational Research, 6(2), 114–118. https://pubmed.ncbi.nlm.nih.gov/24489990/
[19] Shineman, D. W., Basi, G. S., Bizon, J. L., Colton, C. A., Greenberg, B. D., Hollister, B. A., Lincecum, J., Leblanc, G. G., Lee, L. (Bobbi) H., Luo, F., Morgan, D., Morse, I., Refolo, L. M., Riddell, D. R., Scearce-Levie, K., Sweeney, P., Yrjänheikki, J., & Fillit, H. M. (2011). Accelerating drug discovery for Alzheimer’s disease: best practices for preclinical animal studies. Alzheimer’s Research & Therapy, 3(5), 28. https://doi.org/10.1186/alzrt90
[20] O’Collins, V. E., Macleod, M. R., Donnan, G. A., Horky, L. L., van der Worp, B. H., & Howells, D. W. (2006). 1,026 experimental treatments in acute stroke. Annals of Neurology, 59(3), 467–477. https://doi.org/10.1002/ana.20741
[21] Rejuve Biotech stands at the frontline of biotechnological innovation. Leveraging the groundbreaking intelligence of next-gen. (n.d.). Retrieved November 21, 2023, from https://www.rejuve.bio/_files/ugd/6135b4_fbc6ee999bfd45578c39796bc780e0af.pdf